For decades, the basic architecture of remote control nodes consisted of controllers, sensors, local storage, network connectivity interfaces, and batteries. This architecture is widely used in systems controlled by actual operations. In industrial automation systems, the controller monitors multiple sensors at different rates, saves the tagged time sensor data in local or extended memory, and then transmits the data via an industry standard bus such as ProfiBus. In Advanced Driver Assistance Systems (ADAS) or Vehicle Event Recorder (EDR), multiple MCUs can simultaneously capture and control data from automotive electronic systems, providing a superior driving experience and trouble-free data protection. Medical systems have similar applications: critical patient data obtained through sensors will be stored locally or periodically uploaded for centralized storage.
These systems are trying to address the core and fundamental issues in data collection, critical data storage, and action-based action based on data analysis. However, the focus of different systems to solve problems is also different. Industrial systems tend to collect large amounts of data from many different sensors in a very short time interval, while having to keep detailed log records locally and remotely. Automotive system data generation rates are low, but data is of high importance. In some cases, the loss of data may threaten the safety of drivers and passengers. Most cars have a lifespan of more than a decade, so their service life and reliability are a very important consideration when choosing a memory. Portable medical systems focus on power consumption when choosing the ideal data storage. Since implantable medical devices, hearing aids, and the like are all driven by batteries, they are more likely to choose memory with low power consumption and high data storage accuracy. Fault-free data storage with long-term reliability and low power consumption is often a major challenge for system designers to choose storage products.
With the gradual rise of the Internet of Things, all devices are beginning to connect through the network. It is conservatively estimated that 10 billion devices will be connected in 2020, including next-generation products such as automobiles, industrial automation equipment, implantable medical devices, wearable devices and smart homes. The next generation of 5G networks has begun to be deployed in certain regions and is expected to cover most of the traffic generated by these devices. However, data scientists and system designers still have several unresolved issues:
Which devices need to connect to the cloud? How much information needs to be transmitted? How much information can be processed locally? Who will pay for the cloud?
One solution is to upload all the information to the cloud and process the information remotely. But this solution only applies to small and scattered systems. As the world's interconnectedness continues to increase, there will be a large and even excess system for uploading information. In this case, we need to consider the cost difference between the network and the local storage and processing. During driving, an autonomous car will generate several gigabytes of data per hour. So in order to predict future demand, we must now decide which information needs to be transmitted in real time and which can be stored locally so that it can be compressed later. System designers in the industrial and medical fields face the same problem. In the "Industry 4.0" process, the "upload all data to the cloud" approach is gradually turning into a "local processing, smart upload" approach. Therefore, how to choose the most ideal local data storage is extremely important for future system development.
In order to preserve important data, these systems require high reliability, low power consumption, and high security. One way is to use existing flash memory to log data. Flash technology has the ability to read efficiently and has therefore been widely used for boot code and firmware storage. For devices in existing systems, the designer can use flash to record data without having to understand the technical limitations of flash when performing write operations. The flash unit can store new data only if it has been erased beforehand. When programming a flash cell, the logic value can be changed from "1" to "0". In the next upgrade, if the storage unit needs to maintain a logical value of "1", it needs to erase the data. To speed up erase and reduce program time, flash manufacturers have designed various page, block and sector architectures. A page is the smallest unit of data storage that can be programmed into flash at one time. Flash devices have an internal page size buffer for temporary data storage. When the data transfer of the external interface is completed, the device will immediately execute the page program on the erased page in the main array. If the page contains old data, it must be erased before the program starts. The flash cell is degraded each time an erase is performed. This phenomenon is usually quantified as a measure of the durability of flash memory. The most durable flash memory devices can withstand 100,000 erase program cycles, and storage stability cannot be guaranteed after reaching this limit. Although this number looks huge, this number is difficult to meet even the needs of low-end data recording systems.
Some manufacturers use byte programming and push programming from the buffer to the flash. Although this design simplifies program execution within the device, it does not free the flash memory from potential durability limitations. To counteract the above limitations, system designers have been forced to adopt a sophisticated file system to ensure wear leveling of flash cells. The file system software slows down the system.
Imagine a situation where designers consider using a flash-based memory to record data. In industrial automation and asset management systems, sensor nodes capture data at multiple times per second, periodically sample various sensors, and then organize the packet upload network. In general, the number of samples of a packet varies from 16 bytes to 128 bytes. Since the risk of power outages cannot be completely avoided, in order to prevent data loss, designers use non-volatile memory to store data. The vibration sensor or stepper motor position sensor sends pulsed data every few milliseconds, while the temperature or humidity sensor sends data every few seconds, and the data packet stores data for many sensors.
The following chart clearly explains this data. We found that for low-end systems that record 8-16 bytes of data every 1 millisecond, 8 megabytes of flash will be lost in 5 years. However, the depletion period of an automobile or industrial system should exceed 10 years.
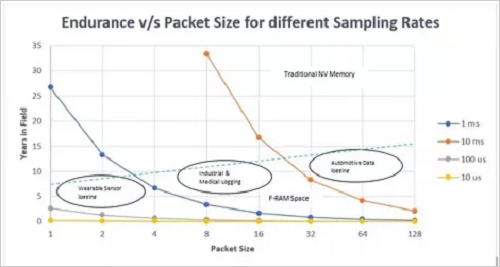
If you use a simple, high-risk approach to adding flash, you need a complex file system to manage wear leveling. If the file system is not deployed, the system needs to periodically perform a chip erase cycle after replacing the entire memory. In today's IoT world, this problem is deteriorating as data collection terminals continue to proliferate. Flash-based memory is ideal for boot code and firmware programs that do not write more than 1000 write cycles during the life of the product. An ideal way to solve this data logging problem is to use high-endurance and non-volatile memory that does not pose a data risk due to program and erase delays. With up to 1014 erase cycles of durability, F-RAM is instantly non-volatile and requires no programming and erase operations to store all data entering the device interface in real time. For example, a 4 megabyte F-RAM storage can process 128 bytes of data stream every 10 microseconds without loss for more than 1,000 years.
F-RAM memory cells consume power only when they are written or read, and standby power consumption is only a few microamps, so F-RAM is the best solution for battery-powered products. F-RAM is suitable for hearing aids with high energy requirements and high-end wearable medical devices for heart rate sampling. In addition, data in automotive systems is continuously entered into memory, and flash-based systems cannot capture data during flash "programming". Only F-RAM based data storage provides high reliability for the system.
F-RAM with near-infinite durability, ultra-low power consumption and instant non-volatility is ideal for important data storage in the connected world.
Aluminum Alloy Rolling Shutter Foaming door
The simple hard fast door sets environmental protection, energy saving, sealing, high efficiency, wind resistance, durability, anti-theft in one. The new metal industrial door type with high-speed partition, the door adopts unique heat insulation structure design, featuring good heat preservation effect, light door body, high strength and fast speed. With this type of door, each time the door is opened and closed, the energy consumption of the enterprise can be saved by about 80%.
The door is widely used in industrial plants in Europe and the United States, easy to use, easy to operate, reliable, and beautiful in appearance. The product is highly reliable, practical, easy to operate and low maintenance costs, and provides a full year warranty after installation. The control system uses the new cutting-edge DSP chip in the field of industrial control as the core, ensuring that there are more than 150,000 fault-free actions per year in the application.
1) Control System
Simple hard fast door adopts special control system, adopts servo special control box, two-phase 220-three-phase 380V-power 0.75-1.5-2.2KW, control box with main power control switch, emergency stop button, manual automatic conversion button , manually control the up and down buttons. Precise control of the opening height of the door.
stainless steel high speed door,roller shutter door , door , Aluminum alloy panel roller shutter door
SHENZHEN HONGFA AUTOMATIC DOOR CO., LTD , https://www.hongfahss.com